A commutator is an electrical switch that periodically reverses the current in an electric motor or electrical generator. A commutator is a common feature of direct current rotating machines. By reversing the current in the moving coil of a motor's armature, a steady rotating force torque is produced. Similarly, in a generator, reversing of the coil's connection to the external circuit produces unidirectional current in the circuit. The first commutator-type direct current machine was built by Hippolyte Pixii in 1832, based on a suggestion by Ampere.
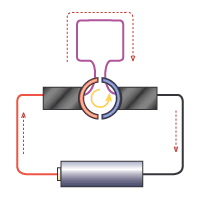
Conventional continuous current flows from the battery. The commutator itself is the red and blue curved segments. The brushes are dark gray and contacting the commutator contacts, and the rotor winding is violet. As the motor rotates, the commutator contacts will turn through 180° and the current flowing in the winding will reverse. The reverse in coil current compensates for the fact that the coil has rotated 180° relative to the fixed magnetic field (not shown). By varying the relative angle between the two fields a torque on the coil can be produced and maintained by the commutator allowing work to be extracted from the coils rotation.
Construction
A commutator typically consists of a set of copper contacts, fixed around part of the circumference of the rotating part of the machine (the rotor), and a set of spring-loaded carbon brushes fixed to the stationary frame of the machine. The external source of current (for a motor) or electrical load (for a generator) is connected to the brushes.
Friction between the copper contacts and the brushes eventually causes wear to both surfaces. The carbon brushes, being made of a softer material, wear faster and may be designed to be replaced easily without dismantling the machine. The copper contacts on small motors (say, less than a kilowatt rating) are not designed to be repaired. On large motors the commutator may be re-surfaced with abrasives, or the rotor may be removed from the frame, mounted in a large metal lathe, and the commutator resurfaced by cutting it down to a smaller diameter.
Each conducting segment on the armature of the commutator is insulated from adjacent segments. Initially when the technology was first developed, mica was used as an insulator between commutation segments. Later materials research into polymers brought the development of plastic spacers which are more durable and less prone to cracking, and have a higher and more uniform breakdown voltage as with mica.
The segments are held onto the shaft using a dovetail shape on the edges or underside of each segment, using insulating wedges around the perimeter of each commutation segment. Due to the high cost of repairs, for small appliance and tool motors the segments are typically crimped permanently in place and cannot be removed; when the motor fails it is simply discarded and replaced. On very large industrial motors it is economical to be able to replace individual damaged segments, and so the end-wedge can be unscrewed and inidividual segments removed and replaced.
Commutator segments are connected to the coils of the armature, with the number of coils (and commutator segments) depending on the speed and voltage of the machine. Large motors may have hundreds of segments.
A practical commutator must contain more than two segments to avoid a "dead spot" where the brushes span both segments, resulting in a short-circuit between them.
Limitations and alternatives
While commutators are widely applied in direct current machines, up to several thousand kilowatts in rating, they have limitations.
Brushes and copper segments wear. On small machines the brushes may last as long as the product (small power tools, appliances, etc.) but larger machines will require regular replacement of brushes and occasional resurfacing of the commutator. Brush-type motors may not be suitable for long service on aerospace equipment where maintenance is not possible.
The efficiency of direct current machines is limited by the "brush drop" due to the resistance of the sliding contact. This may be several volts, making low-voltage direct-current machines very inefficient. The friction of the brush on the commutator also absorbs some of the energy of the machine.
Lastly, the current density in the brush is limited and the maximum voltage on each segment of the commutator is also limited. Very large direct current machines, say, more than several megawatts rating, cannot be built with commutators. The largest motors and generators, of hundreds of megawatt ratings, are all alternating-current machines.
With the widespread availability of power semiconductors, it is now economic to provide electronic switching of the current in the motor windings. These "brushless direct current" motors eliminate the commutator; these can be likened to AC machines with a built-in DC to AC inverter.
The Commutating Plane
In a dynamo, the contact point of where a pair of brushes touch the commutator is referred to as the commutating plane. In this diagram the commutating plane is shown for just one of the brushes.
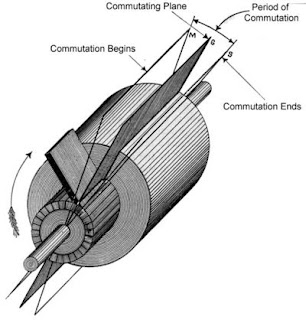
Compensation for stator field distortion
In a real dynamo, the field is never perfectly uniform. Instead, as the rotor spins it induces field effects which drag and distort the magnetic lines of the outer non-rotating stator.
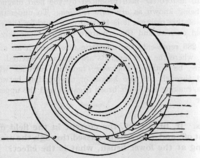
Exaggerated example of how the field is distorted by the rotor.
The faster the rotor spins, the further the degree of field distortion. Because the dynamo operates most efficiently with the rotor field at right angles the stator field, it is necessary to either retard or advance the brush position to put the rotor's field into the correct position to be at a right angle to the distorted field.
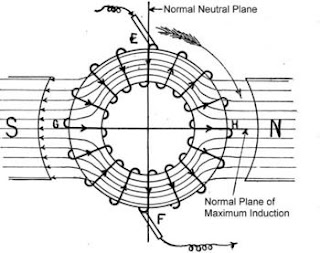
Centered position of the commutating plane if there were no field distortion effects.
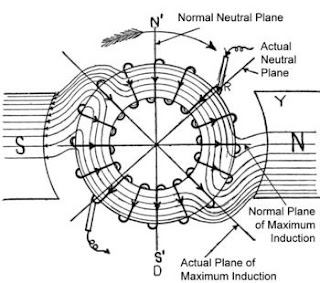
Actual position of the commutating plane to compensate for field distortion.
These field effects are reversed when the direction of spin is reversed. It is therefore difficult to build an efficient reversible commutated dynamo, since for highest field strength it is necessary to move the brushes to the opposite side of the normal neutral plane.
The effect can be considered to be somewhat similar to timing advance in an internal combustion engine. Generally a dynamo that has been designed to run at a certain fixed speed will have its brushes permanently fixed to align the field for highest efficiency at that speed.