In chemistry, activation energy, also called threshold energy, is a term introduced in 1889 by Svante Arrhenius that is defined as the energy that must be overcome in order for a chemical reaction to occur. Activation energy may otherwise be denoted as the minimum energy necessary for a specific chemical reaction to occur. The activation energy of a reaction is usually denoted by Ea, and given in units of kilojoules per mole.
Basically, the activation energy is the height of the potential barrier (sometimes called the energy barrier) separating two minima of potential energy (of the reactants and of the products of reaction). For chemical reaction to have noticeable rate, there should be noticeable number of molecules with the energy equal or greater than the activation energy.
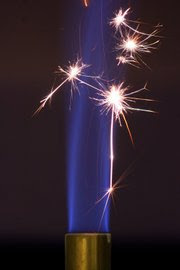
The sparks generated by striking steel against a flint provide the activation energy to initiate combustion in this Bunsen burner. The blue flame will sustain itself after the sparks are extinguished because the continued combustion of the flame is now energetically favorable.
Overview
Main article: Collision theory
Known as the "collisional model", there are three necessary requirements in order for a reaction to take place:
1. the molecules must collide to react.
If two molecules simply collide, however, they will not always react; therefore, the occurrence of a collision is not enough. The second requirement is that:
2. there must be enough energy (energy of activation) for the two molecules to react.
This is the idea of a transition state; if two slow molecules collide, they might bounce off one another because they do not contain enough energy to reach the energy of activation and overcome the transition state (the highest energy point). Lastly, the third requirement is:
3. the molecules must be orientated with respect to each other correctly.
For the reaction to occur between two colliding molecules, they must collide in the correct orientation, and possess a certain, minimum, amount of energy. As the molecules approach each other, their electron clouds repel each other. Overcoming this repulsion requires energy (activation energy), which is typically provided by the heat of the system; i.e., the translational, vibrational, and rotational energy of each molecule, although sometimes by light (photochemistry) or electrical fields (electrochemistry). If there is enough energy available, the repulsion is overcome and the molecules get close enough for attractions between the molecules to cause a rearrangement of bonds.
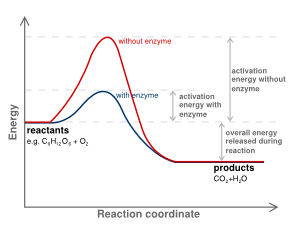
Reaction coordinate showing the relationship between enzyme kinetics and activation energy.
At low temperatures for a particular reaction, most (but not all) molecules will not have enough energy to react. However, there will nearly always be a certain number with enough energy at any temperature because temperature is a measure of the average energy of the system — individual molecules can have more or less energy than the average. Increasing the temperature increases the proportion of molecules with more energy than the activation energy, and consequently the rate of reaction increases. Typically the activation energy is given as the energy in kilojoules needed for one mole of reactants to react.
Mathematical formulation
The Arrhenius equation gives the quantitative basis of the relationship between the activation energy and the rate at which a reaction proceeds.From the Arrhenius equation, the activation energy can be expressed as
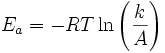
where A is the frequency factor for the reaction, R is the universal gas constant, and T is the temperature (in kelvin). The higher the temperature, the more likely the reaction will be able to overcome the energy of activation. A is a steric factor, which expresses the probability that the molecules contain a favorable orientation and will be able to proceed in a collision. In order for the reaction to proceed and overcome the activation energy, the temperature, orientation, and energy of the molecules must be substantial; this equation manages to sum up all of these things. Because Ea for most chemical reactions is in few electronvolt range (as chemical reactions only involve exchange of outermost electrons between atoms), then raising the temperature by 10 kelvins (at room temperature kT~0.04 eV) approximately doubles the rate of a reaction (in the absence of any other temperature dependent effects) due to an increase in the number of molecules that have the activation energy (as given by Boltzmann distribution equation).
Method of Reducing Activation Energy
Ways in which enzymes can lower the activation energy include: destabilizing the substrate (ground state), or stabilization of the transition state. The enzyme can interact with the substrate to destabilize it via:
geometric interactions
electrostatic (charge-charge) interactions
desolvation
Transition states
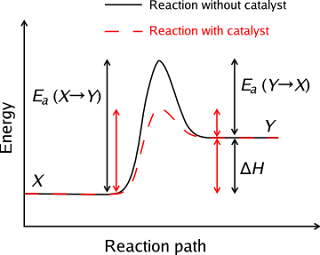
The relationship between activation energy (Ea) and enthalpy of formation (ΔH) with and without a catalyst. The highest energy position (peak position) represents the transition state. With the catalyst, the energy required to enter transition state decreases, thereby decreasing the energy required to initiate the reaction.
The transition state along a reaction coordinate is the point of maximum free energy, where bond-making and bond-breaking are balanced. Transition states are only in existence for extremely brief (10-15 s) periods of time. The energy required to reach the transition state is equal to the activation energy for that reaction. Multi-stage reactions involve a number of transition points, here the activation energy is equal to the one requiring the most energy. After this time either the molecules move apart again with original bonds reforming, or the bonds break and new products form. This is possible because both possibilities result in the release of energy (shown on the enthalpy profile diagram at right, as both positions lie below the transition state). A substance that modifies the transition state to lower the activation energy is termed a catalyst; a biological catalyst is termed an enzyme. It is important to note that a catalyst increases the rate of reaction without being consumed by it. In addition, while the catalyst lowers the activation energy, it does not change the energies of the original reactants nor products. Rather, the reactant energy and the product energy remain the same and only the activation energy is altered (lowered). To further enhance this idea, see this page or the image to the right.
Negative activation energy
In some cases rates of reaction decrease with increasing temperature. When following an approximately exponential relationship so the rate constant can still be fit to an Arrhenius expression, this results in a negative value of Ea. Reactions exhibiting these negative activation energies are typically barrierless reactions, in which the reaction proceeding relies on the capture of the molecules in a potential well. Increasing the temperature leads to a reduced probability of the colliding molecules capturing one another (with more glancing collisions not leading to reaction as the higher momentum carries the colliding particles out of the potential well), expressed as a reaction cross section that decreases with increasing temperature. Such a situation no longer leads itself to direct interpretations as the height of a potential barrier.
No comments:
Post a Comment